From Penn State 14/08/23
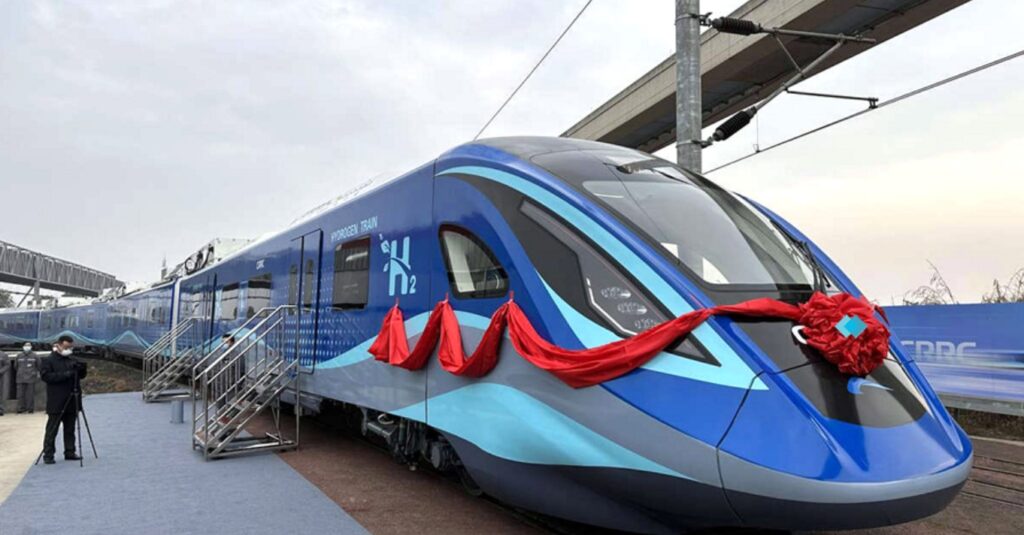
A Penn State-led team of researchers developed a potentially promising approach to make fuel cells more affordable.
The new method reduces the amount of platinum-group metal (PGM) loadings by replicating a process used in computer chip manufacturing.
They published their results this week (July 24) in JACS Au, an open-access journal of the American Chemical Society.
According to corresponding author Christopher Arges, an associate professor in the Department of Chemical Engineering and a faculty member in the Institutes of Energy and the Environment, the new process could reduce the cost barrier to mass producing proton exchange membrane-based fuels cells, which can power heavy-duty vehicles electrically and therefore reduce greenhouse gas emissions.
“Fuel cell electric vehicles are the best electrical power train platform for heavy-duty vehicles and vehicles that constantly need to move goods and people,” Arges said.
The newly developed process involved creating high surface area supports from self-assembled block copolymer templates, which are structures that form into ordered patterns at the nanoscale level.
This process, often referred to block copolymer lithography, is a bottom-up lithographic patterning process used to make dense patterns at feature sizes of 6 to 40 nanometers over large areas.
After making dense high surface area supports with small periodic feature sizes, the team sputtered a thin layer of the PGM on the support substrate.
“We have adopted this technique for the first time to make electrodes with a very low loading of PGM,” Arges said.
“Our new electrocatalysts, which are not alloyed, display competitive performance with state-of-the-art platinum nanoparticles on high surface area carbon supports while showing much better durability when compared to the platinum nanoparticles on high surface area carbon supports.”
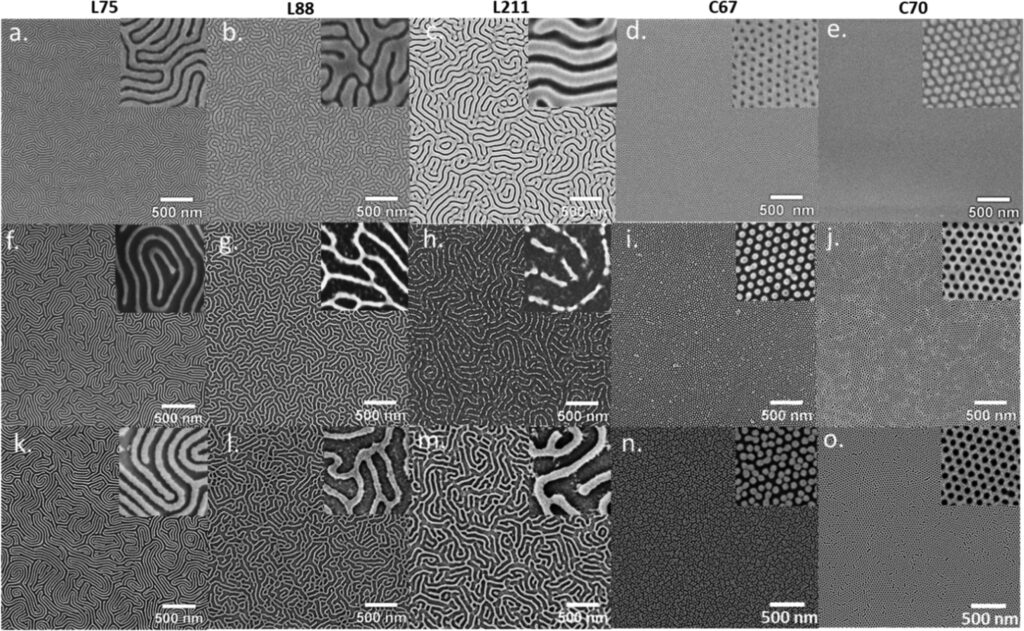
Fuel cell electric vehicles are an alternative to the more common lithium-ion battery electric vehicles.
“Vehicles that use lithium-ion batteries do not perform well when their sole purpose is constantly transporting goods and passengers, such as planes, buses, ships, trains and large trucks.”
“These transport applications do not have time to wait for charging,” Arges said.
“Plus, fuel cell electric vehicles offer high energy density when the vehicle is large and needs to travel a large distance.”
The larger energy density for fuel cell power plants when used in a heavy-duty vehicle allows more energy to be stored within a limited space or weight, which results in longer driving ranges or more efficient energy storage.
Additionally, fuel cell electric vehicles use hydrogen and have no emissions or pollutants.
“The fuel cell does not combust the hydrogen with oxygen in the air.”
“Rather, it performs an electrochemical reaction with hydrogen at one electrode and another reaction with oxygen at another electrode,” Arges said.
“At the oxygen electrode, the protons from the reacted hydrogen are combined with oxygen.”
“Electrons from the reaction move externally through the cell to power the electric motor, and the only byproduct is water.”
“Furthermore, if the hydrogen is derived from water electrolysis powered by renewable energy, it is considered green hydrogen and has zero carbon emissions.”
Even with the appeal of zero emissions and higher energy density, sales of fuel cell electric vehicles lag.
Since 2012, approximately 15,000 fuel cell electric vehicles have been sold in the U.S., compared to 2.5 million battery electric vehicles.
The availability of a hydrogen refueling infrastructure is a limiting factor.
Arges said the team’s new extended surface electrocatalyst can be used for other electrochemical processes involving water electrolysis for making green hydrogen, for which they have a proposal pending.
“Now, we are trying to integrate this process to making membrane electrode assemblies used in actual fuel cell power plants and water electrolyzers,” Arges said.
In addition to Arges, the team included Penn State researchers Deepra Bhattacharya, a Penn State graduate student and the paper’s lead author, and Ke Wang, an associate research professor in the Materials Research Institute.
The U.S. Department of Energy’s Advanced Materials & Manufacturing Technologies Office supported the work upon which this paper was based.